Table of Contents
In Brief
Several studies suggest that dietary supplementation with antioxidants during chemotherapy can influence the response of treatment as well as the development of adverse side effects that results from treatment with antineoplastic agents. Administration of antineoplastic agents results in oxidative stress, i.e., the production of free radicals and other reactive oxygen species (ROS).
Oxidative stress reduces the rate of cell proliferation, and that occurring during chemotherapy may interfere with the cytotoxic effects of antineoplastic drugs, which depend on rapid proliferation of cancer cells for optimal activity. Antioxidants during chemotherapy detoxify ROS and may enhance the anticancer effects of chemotherapy.
For some supplements, activities beyond their antioxidant properties, such as inhibition of topoisomerase II or protein tyrosine kinases, may also contribute to enhancing the antineoplastic action of chemotherapy drugs.
ROS cause or contribute to certain side effects that are common to many anticancer drugs, such as gastrointestinal toxicity and mutagenesis. ROS also contribute to side effects that occur only with individual agents, such as doxorubicin-induced cardiotoxicity, cisplatin-induced nephrotoxicity, and bleomycin-induced pulmonary fibrosis.
Antioxidants can reduce or prevent many of these side effects, and for some supplements the protective effect results from activities other than their antioxidant properties (e.g. glutamine provides nutrients to intestinal epithelium and coenzyme Q10 preserves function of cardiac mitochondria, preventing cardiotoxiciy associated with anthracyline drugs).
Certain side effects, however, such as alopecia and myelosuppression, are not prevented by antioxidants, and agents that interfere with these side effects may also interfere with the anticancer effects of chemotherapy, as interference on this level suggests overriding the anti-proliferative effects of chemotherapy (blood cell proliferation and hair follicle proliferation).
A recent report indicates that approximately 60% of cancer patients use dietary supplements without the knowledge of their oncologist while undergoing cancer treatment. (Prasad 2004)
Does Cancer CELLS Decrease Mitotic Activity?
Question is often raised if cancer cells decrease mitotic activity in the presence of excess free radicals and making them less susceptible to chemotherapy drugs.
Cancer cells have “highly evolved” mechanisms to prevent lipid peroxidation. But excess reactive oxygen species (ROS) (primarily induced by chemotherapy drugs) causes excess oxidative stress which results in lipid peroxidation in cancer cells (as well as normal cells).
This prolongs the G1 phase and may result in cells entering the dormant G0 phase. Thus, excess ROS (“free radicals”) interfere with the cytotoxic effects of antineoplastic agents on cancer cells by interfering with a cancer cell’s progression through its cell cycle.
Tumor cells in G0 are “unaffected” by chemotherapy and can usually reenter the division cycle after chemotherapy is completed. They can also repair damage done by drugs that do not require ongoing DNA synthesis (such as cisplatin).
Since anticancer drugs are effectively ONLY when cells are proliferating rapidly, it stands to reason that oxidative stress, which slows or arrests cell growth, interferes with the effectiveness of chemotherapy. It also explains why slow-growing tumors (such as lung or colon) are relatively unresponsive to chemotherapy.
Oxidative stress, possibly through aldehyde-mediated enzyme inhibition of cyclin-dependent kinases, inhibits transition of cells from the G0 phase (quiescent phase) of the cell cycle to the G1 phase, blocks progression through the restriction point, and causes arrest of the cell cycle at the G1, S, G2, and M phase checkpoints.
For antineoplastic agents that exhibit cell cycle phase-specific activity, e.g., those that interfere with DNA synthesis or block the mitotic process, interference with cell cycle progression may diminish their cytotoxicity.
Even platinum coordination complexes and alkylating agents, which are not considered to be phase-specific agents, require cells to progress through the S phase and G2 phase for apoptosis to occur.
As well, checkpoint arrest also may allow for DNA repair of damage caused by platinum coordination complexes and alkylating agents, and checkpoint abrogation (the opposite of what happens during oxidative stress) has been shown to enhance the cytotoxicity of several types of antineoplastic agents.
By reducing aldehyde generation, antioxidants may counteract the effects of chemotherapy-induced oxidative stress on cell cycle progression and enhance the cytotoxicity of antineoplastic agents.
Can Administration Of Antioxidants Enhances Antineoplastic Efficacy?
As note above, administration of anticancer agents results in a much greater degree of ROS than cancer itself. This high level of ROS during chemotherapy may overcome the antioxidant defenses of cancer cells, which may result in that lipid peroxides reduce or halt cancer cell proliferation and thereby interfere with chemotherapeutic agents.
Therefore, individuals with a relatively impaired antioxidant status are relatively unresponsive to chemotherapy. A number of researchers suggest giving the patient high doses of vitamin E, vitamin C, selenium, beta-carotene, coenzyme Q10 and other selective antioxidants during chemotherapy.
This will prevent the cancer cell from becoming dormant and thus expose it to the cytotoxic effects of the drugs, which enhances the cancer killing effects of chemotherapy drugs
According to Kenneth Conklin, “nutritional therapy with antioxidants during chemotherapy may overcome the growth-inhibiting effects of oxidative stress and maintain responsiveness to anti-neoplastic agents” (Conklin 2000).
Can dietary supplements with antioxidants interfere with the mechanism whereby antineoplastic agents are cytotoxic to cancer cells?
According to Conklin, “This is unlikely, since ROS are not involved in the mechanism of action of most anticancer drugs in current use.” Bleomycin is an exception to the rule. Thus, it may unwise to recommend antioxidants when bleomycin is the chemotherapeutic agent of choice.
Conklin’s article provides not just an excellent review of the data, but a cogent reason why antioxidants are in fact beneficial when used with conventional treatments.
While we await additional evidence from well-designed randomized controlled trials on this topic we can reassure patients that the overwhelming mass of data accumulated so far supports the concurrent use of chemotherapy with dietary antioxidants.
Are there any side effects of ROS on Chemotherapy treatment?
Free Radical Assault Is Not The Means By Which Most Cancer Drugs Kill Cancer Cells, But ROS Does Cause Chemo Side Effects
The drugs of many classes of antineoplastic agents are known to generate a high level of oxidative stress in biological systems. These classes of drugs include the anthracyclines, most alkylating agents, platinum-coordination complexes, epipodophyllotoxins, and camptothecins.
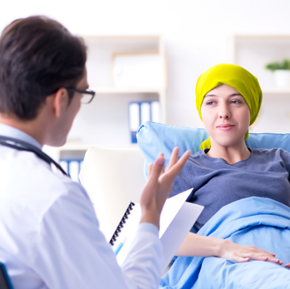
For these drugs, the hepatic microsomal monooxygenase system is a primary site where ROS are generated, although other enzymatic (e.g., xanthine oxidase) and nonenzymatic (Fenton and Haber-Weiss reactions) mechanisms also play a role.
The electron transport system of cardiac mitochondria is another site where significant levels of ROS are generated by anthracyclines .
Although some classes of antineoplastic agents generate high levels of oxidative stress, others, including the taxanes, vinca alkaloids, antifolates, and nucleoside and nucleotide analogues, generate only low levels. Nevertheless, all drugs generate some free radicals.
The mechanism of action of only a few antineoplastic agents has been definitively linked to a free radical intermediate of the parent drug. Examples of antineoplastic agents that have been so linked include mitomycin-C, which creates DNA inter-strand crosslinks following reduction of its aziridine ring, and bleomycin, which cleaves DNA by hydrogen abstraction by its iron-binding arm, which functions as a ferrous oxidase.
Most of the other major classes of antineoplastic agents have well-established mechanisms of action that are independent of free radical intermediates or free radical generation.
These include the antifolates and nucleoside and nucleotide analogues that impact DNA synthesis, the vinca alkaloids and taxanes that interfere with microtubule function, the epipodophyllotoxins (etoposide, teniposide) that interfere with topoisomerase II activity, and the camptothecins (topotecan, irinotecan) that interfere with topoisomerase I activity.
The platinum coordination complexes (cisplatin, carboplatin, oxaliplatin) and most alkylating agents form strong electrophilic intermediates that act via nucleophilic substitution reactions to form inter- and intrastrand DNA crosslinks.
Although toxicity among these agents varies, most side effects also are attributed to nucleophilic substitution reactions, e.g., cisplatin toxicity (nephrotoxicity, neurotoxicity, ototoxicity) is attributable to protein sulfhydryl binding and inactivation of thiol-containing enzymes.
Antioxidants that act as reducing agents do not appear to interfere with the antineoplastic activity of these agents nor do they prevent the development of side effects, which suggests that free radical generation does not play a role in the antineoplastic activity or the toxicity of these agents.
Is Glutathione, NAC and Thiosulfate helpful in chemotherapy treatments?
Glutathione, NAC and Thiosulfate May Not Be The Best Choice Of Antioxidants During Chemotherapy
In contrast to antioxidants that act as reducing agents, nucleophiles, such as glutathione (GSH), N-acetylcholine (NAC), and thiosulfate, can bond covalently to electrophilic compounds such as cisplatin, and mixing cisplatin with thiosulfate prior to administration blocks the antineoplastic agent’s activity .
However, several animal and clinical studies have shown that intravenous administration of GSH shortly before administration of cisplatin reduces the drug’s toxicity without reducing its anticancer activity.
This may be explained by the high renal and neural intracellular levels of gamma-glutamyl transpeptidase (GGT), an enzyme that hydrolyzes circulating GSH (GluCysGly) to Glutamine and Cysteine-Glycine and transports these products into the cell.
The high enzyme levels in normal tissues allow for rapid clearing of circulating GSH, thus preventing cisplatin binding to GSH in the bloodstream, and also results in high GSH levels in renal and neural tissue, thus protecting them from cisplatin toxicity.
Renal cells also have unique mechanisms for concentrating selenium and for formation of methylselenol and glutathionylselenol, compounds that also protect the kidneys from cisplatin toxicity.
Most cancer cells have low levels of GGT. Thus, intravenous administration of GSH does not increase the GSH content of most cancer cells that could reduce the antineoplastic activity of cisplatin. However, GTT may be expressed in higher amounts or be inducible in some neoplastic cells.
The potential selective protection of normal tissues from cisplatin toxicity warrants further investigation.
How Anthracyclines Work (Doxorubicin) – Intercalation Agents?
Anthracyclines chemotherapy are a very commonly used cancer treatment in this day and age. Overall, there is evidence to suggest that concurrent antioxidant administration with these drugs enhances their anti-neoplastic effects, by preventing cancer cells from entering into the G0 quiescent stage. However, administration of these anticancer agents results in a much greater degree of ROS than cancer itself.
This high level of ROS during chemotherapy treatment may overcome the antioxidant defenses of cancer cells, which may result in that lipid peroxides reduce or halt cancer cell proliferation and thereby interfere with chemotherapeutic agents.
Therefore, individuals with a relatively impaired antioxidant status are relatively unresponsive to undergoing chemotherapy treatment. Several mechanisms have been proposed for the anticancer activity of anthracyclines.
Although the most studied anthracycline, doxorubicin, alters membrane function, signal transduction such as pathways involving protein kinase C, and many other cellular functions, the most compelling evidence for its primary mechanism of action is via intercalation with double-stranded DNA and inhibition of topoisomerase II activity.
This effect is evident at clinically relevant concentrations, with the drug being localized primarily in the nucleus of neoplastic cells and acting in the S-phase of the cell cycle. This supports topoisomerase II inhibition as the drug’s primary cytotoxic mechanism.
However, doxorubicin readily undergoes a one-electron reduction to its semiquinone, which can donate an electron to molecular oxygen resulting in superoxide generation.
Although generation of hydroxyl radicals from superoxide is an attractive explanation for the cytotoxicity of doxorubicin, several lines of evidence suggest that this mechanism does not contribute significantly to the drug’s anticancer activity
How Cardiotoxicity From Anthracyclines can be Prevented?
Although free radical generation may not play a significant role in the antineoplastic activity of doxorubicin, there is compelling evidence that disruption of the electron transport system and hydroxyl radical generation in mitochondria of cardiac cells accounts for the drug’s acute and chronic cardiotoxicity.
The selective toxicity of doxorubicin to cardiac cells is accounted for by the unique structure of the cardiac mitochondrial inner membrane, which possesses a cytosolic (outer surface, or intermembranous) NADH dehydrogenase in addition to the matrix (inner surface) NADH dehydrogenase that is present in the mitochondria of all cells.
Doxorubicin (a tetracycline ring with a sugar moiety) is hydrophilic and cannot penetrate the inner membrane and be reduced by the matrix enzyme. However, in cardiac mitochondria, doxorubicin, which can penetrate the outer membrane and enter the mitochondrial cytosol, is reduced by the cytosolic NADH dehydrogenase to its semiquinone.
Intramolecular rearrangement results in formation of the lipophilic deoxyaglycone of doxorubicin that penetrates the inner membrane where it then inhibits coenzyme Q10-dependent enzymes, accounting for disruption of mitochondrial energetics and resulting in the development of acute cardiotoxicity (arrhythmias and reduced ejection fraction).
The deoxyaglycone also competes with coenzyme Q10 (both structurally are quinones) as an electron acceptor, diverting electrons to molecular oxygen with the formation of superoxide radicals, and displaces coenzyme Q10 from the electron transport chain, resulting in elevated plasma levels of coenzyme Q10.
These effects explain the generation of elevated levels of ROS in cardiac cells that lasts for several weeks following administration of doxorubicin and the high levels of mitochondrial DNA adducts that form in heart mitochondria and result in suppression of mitochondrial gene expression for critical components of the electron transport system, such as coenzyme Q10.
These long-lasting effects most likely explain mitochondrial disruption, the first cytological evidence of chronic cardiotoxicity, which leads to myocyte degeneration and cardiac failure.
Preclinical and a limited number of clinical studies suggest that, whereas antioxidants during chemotherapy treatment, in general do not prevent doxorubicin cardiotoxicity, administration of coenzyme Q10 does prevent the development of both acute and chronic cardiotoxicity without interfering with the drug’s anticancer efficacy.
Does antioxidant suppress Apoptosis?
Antioxidants do not suppress apoptosis as apoptosis results from cytochrome c release, which induces free radicals as a result of apoptosis: free radicals do not induce apoptosis
One of the primary pathways of drug-induced apoptosis is the pathway that involves release of cytochrome c from mitochondria.
When cytochrome c is displaced from the electron transport chain, instead of electrons being transferred to oxygen via cytochrome c oxidase with the formation of water, electrons are diverted from NADH dehydrogenase (Complex I) and reduced coenzyme Q10 to oxygen with the concomitant formation of superoxide radicals.
Although superoxide is not highly toxic, mitochondrial superoxide dismutase generates hydrogen peroxide from superoxide and, in the presence of reduced iron that is abundant in mitochondria, and highly toxic hydroxyl radicals are formed via Fenton and Haber-Weiss reactions.
Thus, all drugs that induce apoptosis by this mechanism generate some degree of oxidative stress, although this does not imply that free radical generation is necessary for a drug to exert its cytotoxic effect on neoplastic cells, because the apoptotic process is initiated by cytochrome c release and superoxide generation occurs secondarily.
References
1. Conklin, K. A. (2000) Dietary antioxidants during cancer chemotherapy: impact on chemotherapeutic effectiveness and development of side effects. Nutr. Can. 37:1-18.
2. Lamson, D. W. & Brignall, M. S. (1999) Antioxidants in cancer therapy; their actions and interactions with oncologic therapies. Altern. Med. Rev. 4:304-329.
3. Moss R. The Concurrent Use of Antioxidants and Cytotoxic Cancer Treatments: A Speech to the 7th International Symposium for Biologically Closed Electric Circuits in Biomedicine. July 19-22, 2001. Helsingør, Denmark.
Specific Antioxidants of Choice:
Vitamin C – studies support vitamin C daily dosages in the range of 2,000-5,000 mg (Pauling suggests up to 10,000 mg per day – as dictated by bowel tolerance (Prasad 1999)
Vitamin E Succinate – studies support daily dosages in the range of 2,000-3,000 IU
Beta-carotene – studies support daily dosages in the range of 50,000-100,000 IU
Selenium – studies support daily dosages in the range of 600-800 mcg
Coenzyme Q10 – studies support daily dosages in the range of 150-300 mg
Preliminary human studies have shown that the above antioxidants can improve patient outcomes when used in combination with chemotherapy drugs in cases of various types of cancer (small-cell lung cancer; breast cancer stage 0-III, ovarian cancer) – (Prasad 2004). As a single agent Beta-carotene has been shown to reverse oral leukoplakia, likely via its effects on cell differentiation. (Prasad 1999)
Studies suggest that the above antioxidants can reduce recurrence of breast cancer in patients who were previously treated with conventional therapies. (Lockwood 1994; Fleischauer 2003)
Glutamine – glutamine has been shown to enhance the antitumor effectiveness of methotrexate in laboratory animals and reduce the bacteremia and mucosal injury associated with entercolitis of rats given methotrexate. Human studies suggest that glutamine protects the intestinal tract from damage from a variety of chemotherapy drugs. A typical schedule is 6 gm, three times per day (total of 18 grams), beginning three days prior to onset of chemotherapy administration.
Genistein – Genistein inhibits DNA topoisomerase I and II in a similar fashion as the chemodrugs doxorubicin and etoposide – via enhanced topoisomerase IImediated DNA cleavage, resulting in cell cycle arrest. Genistein also inhibits the binding of ATP to its binding site on topoisomerase, which also inactivates the enzyme – These effects lead to inhibition of DNA replication and subsequent antiproliferative effects. (Conklin 2000)
Quercetin – Like genistein quercetin is able to inhibit DNA topoisomerase II, thereby shutting down cancer cell proliferation in experimental studies
References